Hello everyone! Some people have asked me to post about my opinion on the recent claims that gravitational waves have been observed. There are a lot of things about these discoveries worth pointing out in such an informal arena as this one, so sure why not. I am partially adopting an earlier post on this topic, so my apologies for any disjoint trains of thought.
First off, lets begin by pointing out that these claims are sensational. Really amazing! To claim observation of something as exotic as merging black holes, using a new kind of exotic sensing device which has never detected anything before, is nothing short of sensational.
You may have surmised by now that I have been somewhat skeptical about these claims. This is really the only move available to a scientist. One has to be skeptical. This is called being a scientist. If you are religious or a fanatic, you will believe what people tell you based on their clothing, reputation, or credentials. There is a well known logical fallacy known as “appeal to authority”. If you are a scientist, you will question what they tell you and try to come up with your own experiments or models to verify or confirm what they told you. To a scientist, a Nobel prize awarded to some theory or another is a reason to pay attention to that theory – a good reputation is a reason to take a look. However it says nothing about the validity of the theory or observations. Those must come with understanding and experiment.
Big Science
This leads us to the general conundrum of what they call “big science”. This kind of science includes all experiments that are expensive or difficult enough that they can’t be repeated easily. For traditional science, repeating an experiment is the very heart of progress and the scientific method. However, for these “big science” experiments, we cannot so do. Does this mean we should reject all results from big science? Not at all. It just means that the science is a work in progress. It means we are waiting for the next round of experimenters to verify the results, and it means that we still have work to do. Good stuff!
Gravitational Waves – We all knew they are real
OK so on to the gravitational waves. The first thing that needs to be said is that gravitational waves are real. There is no denying this. Gravity is, no matter what formulation you prefer, capable of producing a force, or at least one that looks like a force to observers. This force is at least somewhat related to the direction of a source. Therefore, if we move the source – we have changed the force field. This change in force field will propagate outwards and we can call it a gravitational wave. For the electromagnetic force, this is what we call light – exactly that thing that happens when we accelerate a charge. All light of all frequencies is created by acceleration of charge in exactly this manner. Similarly, we know that moving an active gravitational source (like any mass) we will change the force field and produce a gravitational wave. At question here is not whether gravitational waves exist, we know they do. There is no way they couldn’t. What is at question is the details about how they can be measured with what apparatus, and how strong they are – and what objects have created them. Different types of General Relativistic theories might produces certain different magnitudes of waves for example, while a modified Newtonian theory might produce others. Different accelerated sources will also of course produce different signatures in gravitational radiation. What kind of acceleration of masses exists around us to observe is another question. The speed of propagation is another interesting question, and one that remains to some degree open. We won’t discuss it further here but interested readers are recommended to look at the excellent papers debates by Steve Carlip and Tom Van Flandern.
OK lets get on to some problems with the LIGO experiment and its ilk. The biggest problem in my mind is that the experiment is uncalbibrated. To somebody who has worked on scientific instruments, this is shocking. However, it may be unsurmountable. The problem basically is that the instrument has not looked at any control source. Consider for example Galileo’s first telescope. To see that it worked, he focused on a far off mountain or a ship. This enabled confidence in his new device, which only then did he point towards the heavens. To have trusted some device pointed at the heavens when nothing else had ever been viewed through it, well this is what we are told to do by the authors of the LIGO papers. Particle detectors are calibrated with known particle beams, just as voltmeters are calibrated with known voltages to assure that they are accurate. So, is it possible to calibrate these detectors with a local source?
Calibration attempt: drop a 100 kg mass onto the ground
Lets look at the gravitational radiation which this will create and compare it to the radiation from the source claimed by Abbott et al. Sure, a 100kg object is really massive, but we’re talking big science here.
First off there is the difference in mass. The 100 kg test mass is

times smaller than the 30 solar mass black hole. That’s going to make a lot less radiation right? Well yeah. Especially because the radiated power is proportional to the
square of the source. That’s a factor of

.
How about the distance? Well a light year is
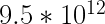
km. 1.3 billion light years is therefore
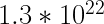
km. This supposed black hole merger event is
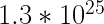
times further away than our test mass dropping on the ground one meter away. Oh, and don’t forget that the intensity of the radiation falls off as the
cube of the distance from dipole sources. (Edit: gravitational radiation is always quadrupolar so the field may fall off even faster. However some have argued that the power falls of as the square of the distance, and that this power is directly measured by the LIGO device, which if true would change the conclusions here). The intensity attenuation from our black hole merger due to distance from source will be a whopping
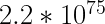
times larger than the attenuation of radiation from our test mass.
The only thing missing from our back of the envelope calculation here is the acceleration applied to the mass. After all, it is acceleration of a source which causes radiation. A stationary charge does not light make, nor does a stationary mass emit gravitational waves. For the test mass, lets assume we are going from 10m/s to 0m/s in 0.001 seconds when the thing hits the ground (because it’s soft ground, the test mass is soft, and it takes some time to stop the test mass). That’s an acceleration of 10000 m/s/s or about 1000 Gs (commercial crash test accelerometers go to 2000Gs or higher).
For the black holes it isn’t too hard to put an estimate on the acceleration. The Schwarzchild radius of the black holes they report as about 210 km. This means when they touch, the centers of mass will be 420km apart. Using this one estimates using the Newtonian limit of about twenty billion m/sec/sec or 2 billion Gs:
Buckle your seatbelt!
It’s the square of the acceleration which determines the emitted power from an accelerated source, thus our difference between the falling test mass and the black hole will be a factor of
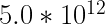
.
–
–
–
That’s it! OK lets recap the three relevant differences between our emitting systems and see how we did:
–
A) Source magnitude squared- Black holes ~

times larger
–
B) Attenuation – Black holes ~
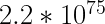
times smaller
–
C) Acceleration squared – Black holes ~
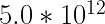
times larger
–
Add it up and what do you get? The gravitational radiation from the test mass hitting the ground could be about 1200 times stronger than the radiation from the distant alleged black hole merger.
Granted, this is a back of the envelope calculation. The proper calculation of acceleration and radiation will take into account relativistic effects and precise orbital mechanics. Stopping our massive test mass in a millisecond might not be possible. But these are second order effects and the point remains: terrestrial sources of gravitational radiation should be visible if such distant sources are also visible. Just imagine that you could drop much more massive object than 100kg, such as an asteroid. Accelerations could also be larger than the 1000 Gs estimate. A landslide might not be a great calibrated source but clearly would be a strong emitter. How about 100 cars all colliding with a reinforced concrete wall simultaneously? All in the name of science of course. 100 not enough? Let’s make it 1000 in the second round of proposals. CMEs might be a perfect test.
EDIT: the number one comment on this discussion is that in the far field we don’t really attenuate our radiation as fast as the cube of the distance. If we assume that our detector is capable of detecting a variation in g field with amplitude which decreases only as the square of the distance, then it appears we are still some 20 orders of magnitude shy of reaching a similar signal with our test mass! However this neglects the fact that most of the attenuation will occur before we reach the transition zone to the far field (“Fraunhofer region”), when our distance from the source becomes sufficiently far that we can ignore any internal dynamics. What happens here is that the power in the near field falls off as the 4th power of distance (see MWT “Gravitation” for an explanation of why gravitational radiation is all quadrupolar). If we take ~50 km as the wavelength of the radiation, then a rough estimate of this transition zone will be 100 km away from the source. This provides a factor of 10^5^-4 = 10^-20 in attenuation of signal before we reach the far-field zone! In other words, for the purposes of this estimate, we are very similar in our predication of amplitudes between our very nearby test mass and our extremely distant black hole merger. Note that an even more simple estimate of total radiated power, followed by an assumption of spherical symmetry, does not capture the real attenuation due to the multipole nature of the radiation. Our improved estimate leaves us with one order of magnitude lower signal from the test mass, in other words – we can multiply our test mass by 10 on increase the acceleration on it by 10 to match the signal of black hole mergers.
Coincident with Gamma Ray Burst
On Sept. 15, 2014, a LIGO signal was observed and coincident with this signal a gamma ray pulse was observed by the Fermi gamma ray telescope. (!!!) This was too close for coincidence, appearing 0.4 seconds later and the team calculated a 0.22% probability of such an event occurring randomly. If this were bright enough it would alone confirm that LIGO was seeing something important and real (despite the lack of calibration). Unfortunately the team from INTEGRAL gamma ray telescope put a damper on this sensational claim by putting a low upper limit on the gamma ray emission (they didn’t see a serious burst). Another team re-analyzing the Fermi data concluded that there wasn’t much of a burst there either. The jury is still out, for a review of the debate see https://arxiv.org/abs/1801.02305.
Conclusions
I am a huge fan of interferometry science and the LIGO and VIRGO projects. However we should be careful to jump to any conclusions at this stage of Big Science. I expect that future gravitational wave observations will have something to say about the early conclusions here, and I especially await any team that can observe the radiation from a more local source, an asteroid impact or a CME or even a laboratory source could fit the bill. Until then I would suggest more cautious language such as “we may have seen black holes merging”, or “signals consistent with black holes merging” as this exciting field isn’t to a high enough level to rule out other sources for the observed signals.
EDIT 2)
There seems to be some pushback on the signal processing methods used to pull out the signal in the alleged observations:
https://arxiv.org/abs/1711.07421
It could also be that other known sources (other than CMEs, impacts mentioned earlier) could be used for calibration:
https://www.space.com/white-dwarf-binary-gravitational-wave-source-discovery.html